Appendix D. Zero-Emission Vehicle Resources
- D.1 State DOT Support for EV Deployment
- D.2 Potential Demand for EVs
- D.3 Emissions Benefits of EVs
- D.4 Fuel Sales and Revenue Implications of EVs
- D.5 Hydrogen as an Alternative Clean Fuel
- D.6 Works Cited
Electrification of transportation vehicles and equipment has emerged in recent years as what is most likely to be the largest source of greenhouse gas (GHG) emission reductions. While State departments of transportation (DOTs) do not have authority over all of the policy mechanisms to encourage and accelerate electric vehicle (EV) adoption, they are important partners, working with other State agencies, municipalities, and the private sector to provide infrastructure and incentives and develop policy supporting EVs. This appendix provides resources for those efforts.
D.1 State DOT Support for EV Deployment
Internationally, EVs have proliferated in the past decade, increasing from virtually zero sales in 2010 to over 2 million per year by 2018 [International Council on Clean Transportation (ICCT), 2019a]. This rapid growth has been driven by declining costs and targeted policy actions. Agencies looking to establish public policy to facilitate EV deployment should focus on several key areas that research indicates generate the highest EV adoption rates: consumer communication and education efforts; efforts to achieve cost parity, including long-duration rebates; and access to high-occupancy vehicle (HOV) and high-occupancy toll (HOT) lanes [Fordham, Norris, and Proudfoot, 2015; Erdakos et al., 2019]. Of these areas, State DOTs are best positioned to assist with communication/education and HOV/HOT lane access. They can also play a role in the deployment of public charging equipment and facilities.
D.1.1 Infrastructure
Consumer demand for EVs is closely linked to the availability of Electric Vehicle Supply Equipment (EVSE). Governments, including State DOTs, can take a leading role in the provision of these facilities. State DOTs can work as a partner in developing resources for municipal and regional agencies to incorporate EVSE requirements and incentives into regional plans, building codes, and zoning ordinances. They may also be partners in opening public utility commission discussions to consider deployment approaches and convening stakeholders to establish a framework for EVSE interoperability and permitting [Zero Emission Vehicle (ZEV) Task Force, 2018]. For example, local and State governments can require that chargers, or wiring to accommodate them, be integrated into building plans, or that a certain percentage of parking spots allocated to new buildings be reserved for plug-in electric vehicles (PEVs) (ICCT, 2019a; Zhu and Nigro, 2013).
The availability of EVSE will determine the useful range of EVs and is thus crucial for addressing consumers’ “range anxiety.” States have recognized the important role of EVSE in electrification efforts; already, 35 States have opted to spend the maximum allowed share of their Volkswagen emissions settlement funds, 15 percent, on EVSE (Rogotzke, Eucalitto, and Gander, 2019). There will be significant demand for this infrastructure in the near future. One analysis estimated the need for EVSE to accommodate the EVs in the largest utility service territories in 12 States. Results indicate that depending on the electrification scenario, 2.6 to 17.5 million home chargers and 121,000 to 754,000 public chargers will be required by 2035 to accommodate 2.9 to 19.1 million EVs. Under the latter scenario, the estimated cost is $2.7 billion (M. J. Bradley & Associates, 2017). Another model estimates the number of nonresidential chargers needed in 2050 under three scenarios as shown in Table D.1.
Table D.1 U.S. Charging Infrastructure Required for Various Electrification Scenarios
Electrification Scenario | Direct Current Fast Charger | Nonresidential L2 | |
Stations | Plugs | Plugs | |
Reference | 17,000 | 55,000 | 1,200,000 |
Medium | 106,000 | 343,000 | 6,110,000 |
High | 138,000 | 447,000 | 9,980,000 |
Source: Mai et al., 2018.
Most charging already is done at home, and it is likely that EV drivers will continue home charging in the future. At the same time, EVSE locations are important for overall convenience and accessibility, particularly in urban areas where drivers may not have charging infrastructure at home. Suitable public local destinations for installation must be locations where people spend multiple hours or stay overnight, such as malls, hotels, parks, park-and-ride parking lots, and tourist attractions. Workplaces may also encourage EV adoption through proactive installation of charging infrastructure [ICCT 2019a, Transportation Research Board (TRB)/National Research Council (NRC), 2015].
Charging stations may operate under subscription-based, pay-as-you-drive, or free charging models, and governments may also seek to encourage installation by private companies through direct financial incentives. Utilities, too, can accelerate deployment through the provision of public charging infrastructure and by supporting private EVSE installation (Fordham, Norris, and Proudfoot, 2015; M.J. Bradley, 2017).
The U.S. Department of Energy Alternative Fuels Data Center provides information on the locations of EV charging stations.
D.1.2 Information
Public agencies will play a crucial role in expanding consumer awareness and understanding of EVs. Governments can help address information gaps that act as barriers to adoption, including “range anxiety” and misperceptions around charging; understanding of electricity consumption; calculation of fuel costs; and a lack of clear information on consumer benefits (TRB/NRC, 2015). Agencies can support the development of consumer-driven websites with information on buying an EV, charging behavior, and consumer dividends (Zhu and Nigro, 2013). Public “ride and drive” events and EV showrooms may also bolster the consumer experience alongside more traditional advertising and social media channels (ZEV Task Force, 2018). A State DOT can either lead or support education and outreach efforts.
D.1.3 Incentives
Research suggests that HOV/HOT lane access is an important step for States looking to encourage the purchase of EVs. Free or reduced access to these lanes helps shift the economic calculus of an EV purchase, and type of access can be tailored to geography. In California, for example, zero-emission vehicles receive free or discounted entry to express lanes in some cities. In China, some cities exempt EVs from areawide license plate-based driving restrictions (ICCT, 2019a). State DOTs would play a lead role in setting and enforcing HOV/HOT lane access policies. It will be important to balance the desire to encourage EVs with the need to maintain HOV/HOT lane traffic speeds to serve their intended purpose. Access policies may need to set phase-out or sunset clauses to limit access in the future as EV market penetration reaches a certain level.
State DOTs typically would not play a lead role in setting policy for EV financial incentives, although the registry of motor vehicles (if part of the DOT) may play a role in administering some types of incentives. However, they can provide information to support the establishment of incentives by including these as part of GHG strategy analysis for the transportation sector. Several financial levers are available. States may consider rebates, income tax credits, sales and excise tax exemptions, and other consumer purchase incentives as well as how existing programs might be expanded to reach more or different types of consumers (ZEV Task Force, 2018). Some research has indicated that the existing Federal income tax credit, for example, would prove even more effective in encouraging the purchase of EVs converted to a rebate at point of sale. In general, EVs have proliferated only in markets where financial incentives are able to reduce the upfront cost of the vehicles (TRB/NRC, 2015; ICCT, 2019a).
D.1.4 Direct Deployment
Finally, DOTs can lead the way in deploying EVs. These may include light-duty vehicles for the DOT’s fleets, providing funding and technical support to public transportation agencies to electrify buses, and purchasing electric or hybrid-electric medium and heavy-duty operations and maintenance equipment as it becomes available meeting vocational requirements. Taxi, ride-hailing, and car-sharing fleets offer high operational savings due to their higher mileage, and the DOT may be a partner with other State and municipal agencies designing incentives and fee structures to encourage electrification in these sectors. States can advance the electrification of public fleets through integrating EVs into procurement processes, pursuing relevant Federal tax credits, and setting long-term statewide goals for electrification (ZEV Task Force, 2018).
D.2 Potential Demand for EVs
To date, the rapid growth of EVs worldwide has been driven by technological advances, with battery cost the most significant. Based on the trajectory of battery costs alone, for example, the price of EVs is expected to decrease by $7,000 to $9,000 from 2020 to 2025 (ICCT, 2019a). Market penetration rates will in the long term represent a confluence of not only product technologies but charging infrastructure availability and the impact that these and other factors have on consumer attitudes regarding cost, reliability, and performance of EVs (TRB/NRC, 2015; Erdakos et al., 2019; Fordham, Norris, and Proudfoot, 2015). There is substantial uncertainty around these variables; as such, estimates of anticipated fleet penetration vary widely. Examples of studies and data sources looking at potential market penetration scenarios include the following:
- U.S. Department of Energy (DOE) Annual Energy Outlook (AEO)—This data source provides national estimates of future market penetration (percent of sales, stock, and energy use) for a variety of light-duty vehicle technology types, including electric and plug-in hybrid electric vehicles of varying ranges. Estimates are provided for Reference Case, High Oil Price, and other scenarios and are based on the National Energy Modeling System (NEMS). AEO’s estimates of electrification are generally viewed as conservative, with the 2019 AEO Reference Case showing a light-duty market penetration (vehicle stock) of just under 10 percent in 2040.
- NCHRP Web-Only Document 274: Zero Emission Vehicles: Forecasting Fleet Scenarios and their Emissions Implications (Erdakos et al., 2019)—This report uses the Oak Ridge National Laboratory MA3T model to develop EV market penetration scenarios based on various assumptions about infrastructure expansion, incentives, and vehicle costs. Estimates range from a low of 9 percent to a high of 38 percent of the light-duty vehicle population in 2040. The report also estimates the GHG and criteria pollutant reductions associated with different levels of market penetration.
- National Renewable Energy Laboratory Electrification Futures Study (Mai et al, 2018)—This study includes low, medium, and high scenarios of EV market penetration ranging from 11 to 84 percent in 2040 for light-duty vehicles. Scenarios are also provided for medium and heavy duty trucks.
- FHWA, Feasibility and Implications of EV Deployment and Infrastructure Development (Fordham, Norris, and Proudfoot, 2015). This report, in addition to looking specifically at the role DOTs can play in EV deployment, develops eight scenarios of EV deployment based on a variety of research reports and public and private sector publications. Estimates range from less than 10 million to over 100 million EVs nationwide in 2040.
Private sources also provide their own forecasts. These forecasts are continually evolving as the state of technology and the consumer market evolves. Analysts should check for the latest available information while also recognizing the significant uncertainty that underlies any forecast of EV deployment at the present time.
D.3 Emissions Benefits of EVs
EVs produce zero tailpipe GHG emissions and therefore are not typically included as part of transportation mobile-source emissions inventories. However, GHG emissions are produced as a result of electricity generated to power the vehicle. The GHG intensity per mile of EV travel will be a function not only of the efficiency of the vehicle itself, but also of the carbon intensity of the electricity generation grid from which the vehicle is charged. This may even vary by time of day depending upon the power sources in use for peak versus off-peak load generation. Emissions from electricity generation will be captured in inventories for the electric power sector; however, it is often useful for evaluation purposes to understand the amount of emissions associated with transportation users of electricity.
The following procedure can be used to estimate GHG emissions from EVs:
- Determine the average fuel efficiency (in miles per gallon) of a conventional fuel (gasoline or diesel) vehicle most closely corresponding to the type of EVs to be evaluated—e.g., light-duty auto/truck, medium truck, heavy truck, bus. In this example, an average light-duty vehicle efficiency of 23.8 miles per gallon of gasoline is assumed (“light duty stock” in 2019, based on the AEO 2019 Reference Case).
- Convert miles per gallon to British thermal units (Btu) per mile. This example uses an energy content of 120,286 Btu per gallon gasoline with a 10 percent ethanol blend.[D-1]
- 1 / [23.8 mi/gal / 120,286 Btu/gal] = 5,054 Btu/mi
- Convert Btu per mile to megajoules of energy (MJ) per mile using an energy conversion factor of 948 Btu/MJ.
- 5,054 Btu/mi / 948 Btu/MJ = 5.33 MJ/mi.
- Divide by the energy efficiency ratio (EER) of an electric to conventional fuel vehicle. This ratio is generally estimated to be in the range of 2.5 to 3.0 for light-duty vehicles. Recent studies of heavy-duty vehicles, including trucks and buses, have estimated ranges of 3.0 to 4.0 (c.f. CARB, 2017). This step accounts for the greater efficiency of an electric drive vehicle compared to a conventional fuel vehicle.[D-2]
- 5.33 MJ/mi / 3.0 = 1.77 MJ/mi.
- Multiply by the carbon intensity of the state or regional electricity grid. This may be obtained for the most recent reported year from the U.S. Environmental Protection Agency (EPA) eGRID database. For example, the average rate for Virginia in 2018 is estimated to be 93.6 grams CO2 per MJ of electricity consumed. Adjustments may be needed for future year analysis based on policy-set or modeled reductions in the carbon intensity of the electricity grid.
- 1.77 MJ/mi * 93.6 g/MJ = 166 g CO2/mi (compare with 370 g/mi for the gasoline vehicle, converted at a rate of 8,000 g CO2 per gallon gasoline).
- Multiply by the VMT of electric vehicles to get total CO2 emissions.
Plug-in hybrid EVs are somewhat more complicated to analyze, as their emissions are split between on-board petroleum consumption and grid-generated electricity. The fraction of distance driven in gasoline versus all-electric mode must first be estimated. Then, the efficiency in each mode can be used to estimate energy consumption and GHG emissions by fuel type. Lacking other data, a reasonable assumption may be that gasoline-mode efficiency is similar to an internal combustion engine vehicle and electric-mode efficiency is similar to an EV.
Depending on the policy context for regulating fuel efficiency, there may not be a one-to-one correspondence between EVs sold and overall GHG benefits. Under the Federal Corporate Average Fuel Economy (CAFE) standards, for example, the GHG benefits of EV (or other alternative fuel vehicle) sales could be offset by sales of less-efficient vehicles, with the overall sales mix still achieving the same corporate average level. In the long term, however, policy designs that incentivize EVs may pay off by bringing technology to scale that makes it easier to achieve more stringent fleet-average targets. For more discussion of the relationship among Federal standards, EVs, and GHG benefits, see Jenn, Azevedo, and Michalek (2016).
D.4 Fuel Sales and Revenue Implications of EVs
The transportation infrastructure in the United States is funded in large part by the motor fuels tax, and there is a persistent notion that EV owners, in driving more efficient vehicles, do not pay to support their use of transportation infrastructure. One response to this idea has been to charge EV owners a supplementary fee; as of September 2019, 28 States had adopted added registration fees for EVs. However, research indicates that adding fees for EVs to make up the difference is unlikely to compensate agencies for the ongoing, and much larger, decline in fuel tax revenues associated with more efficient combustion vehicles. Further, these fees send mixed messages to consumers and disincentivize the purchase of EVs. Instead, shifting to user-pay policies such as a vehicle miles traveled (VMT) fee, tolls, or other road user charges may in the long term offer more sustainable funding solutions (ICCT, 2019b; Rogotzke, Eucalitto, and Gander, 2019; TRB/NRC, 2015). Moreover, EVs are not likely to have a significant impact on revenue until their market penetration reaches significant levels (e.g., 5 to 10 percent of vehicles on the road), which is not expected to happen until at least the 2025 to 2030 time frame.
D.5 Hydrogen as an Alternative Clean Fuel
D.5.1 Market Potential for Hydrogen versus Battery EVs
Hydrogen fuel cells are a form of electric drive powered by hydrogen stored on board the vehicle, rather than batteries. The vast majority of hydrogen today is produced through fossil-fuel reforming, a process that produces a reaction between natural gas and steam. Hydrogen can also be produced from renewable sources, using biogas, a gaseous form of methane obtained from biomass, or through electrolysis using electricity generated by renewable sources.[D-3]
Both hydrogen fuel cell and battery EVs are being advanced as potential “zero emission” vehicle technologies. California’s Zero-Emission Vehicle (ZEV) program, which requires auto manufacturers to offer for sale specific numbers of the very cleanest cars available, includes full battery-electric, hydrogen fuel cell, and plug-in hybrid-electric vehicles among its designated clean technologies.[D-4]
In the light-duty market, most of the current focus is on electrification rather than fuel-cell electric vehicles (FCEVs). As of 2019, there were three light-duty FCEVs on the market in the United States, and only in select states; approximately 18,000 FCEVs had been sold or leased globally, compared with the millions of battery-powered EVs sold annually worldwide.[D-5],[D-6]
There are several reasons for the proliferation of battery-powered EVs over FCEVs:
- Technology readiness: Battery drive technology has a higher state of readiness, while the fuel cell industry is still determining how to manage several existing reliability issues. For example, repair and maintenance costs of fuel cells can lead to a substantial cost increase for end users, reducing the ability to widely commercialize the technology.[D-7]
- Fueling/charging infrastructure: There is a substantial existing network of electric charging infrastructure in the United States, in addition to ubiquitous opportunities for low-speed home charging, whereas the lack of a similar network for hydrogen vehicles in all states but California has effectively limited use of hydrogen-powered vehicles to that state (U.S. Department of Energy, Alternative Fuels Data Center. Alternative Fueling Station Locator. https://afdc.energy.gov/stations#/find/nearest, Accessed August 2020.). There are ongoing challenges related to using the stations that do exist. Generally, the same station cannot serve cars and heavy-duty vehicles due to the vehicles’ differing fueling protocols. Some users also experience issues around fuel cell nozzles becoming frozen. The two companies that manufacture most nozzles used in California are working with automakers and station developers to find solutions.[D-8] Creating a new network for hydrogen-powered vehicles would require substantial new investment. As the network grows, though, developers may be able to achieve economies of scale; one report found that capital costs for hydrogen infrastructure could be reduced by 50 percent through economies of scale by as early as 2020 if developers were to build between 30 and 50 hydrogen stations per year globally.[D-9] In Figures D.1 and D.2, the U.S. Department of Energy illustrates both the lack of hydrogen charging infrastructure and the proliferation of electric charging stations in the United States.
- Resource cost: Electricity is less expensive than hydrogen. Most hydrogen produced today is produced through electrolysis based on natural gas feedstock, with typical prices around $16 per kilogram in California, equivalent to over $5 per gallon of gasoline on an energy basis (CEC and CARB, 2019).
Figure D.1 Hydrogen Fueling Stations in the United States
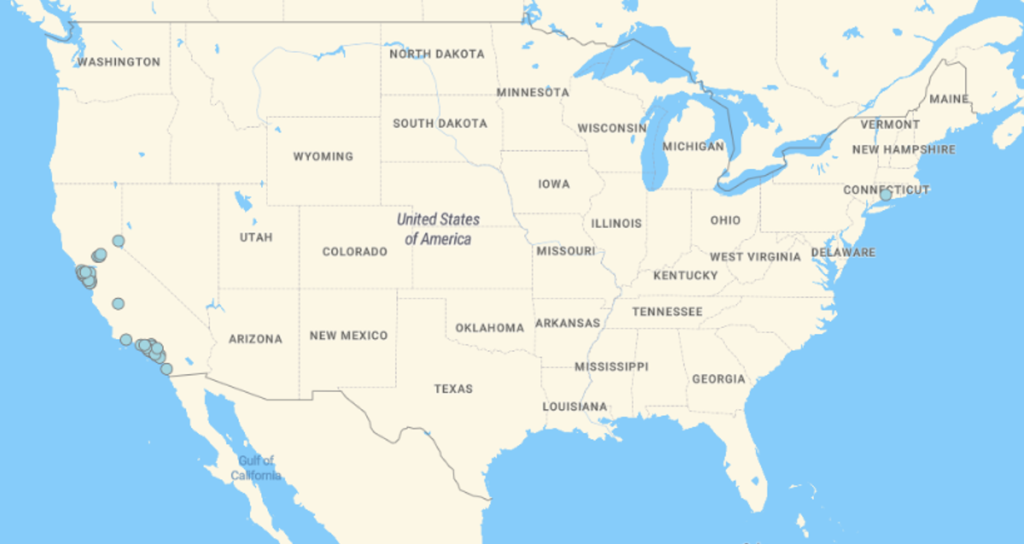
Figure D.2 Electric Vehicle Charging Stations in the United States
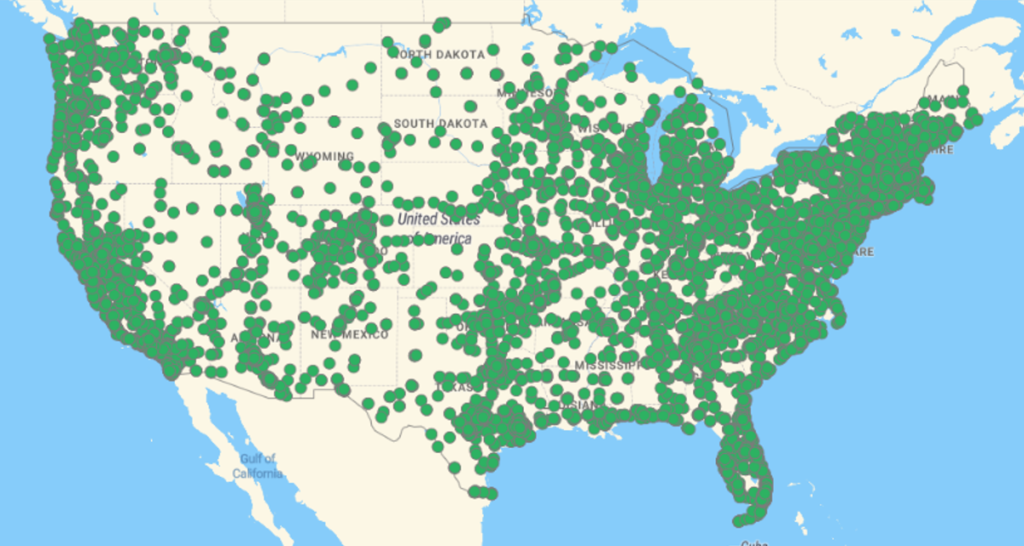
Source: U.S. Department of Energy, Alternative Fuels Data Center. Alternative Fueling Station Locator. https://afdc.energy.gov/stations#/find/nearest, Accessed August 2020.
At the same time, hydrogen fuel cells offer several advantages over EVs, including a higher density of energy storage, the ability to refuel in a matter of minutes, and range; most FCEVs have a range of 200–300 miles, which is approaching that of light-duty gasoline-powered vehicles. This means FCEVs may still be the most viable zero-emission technology for certain market segments such as long-haul trucks. Studies show a wide range of potential market penetration scenarios for different technologies in the medium and heavy truck sectors, depending on assumptions about fuel costs, infrastructure investment, technology development, etc.
National Center for Sustainable Transportation research has examined the extent to which battery-electric and fuel cell technologies will proliferate in the medium-duty and heavy-duty vehicle markets. The research found that to be competitive with corresponding diesel-powered vehicles, the unit cost of a fuel cell system must be $80-100/kilowatt (kW) (the comparable figure for EV batteries is also $80-100/kW). The research also found that the long-term economics of battery-electric buses and trucks look more favorable than that for the fuel cell/hydrogen option if range requirements can be met using batteries. This is primarily due to the significantly lower energy operating cost ($/mi) using electricity rather than hydrogen.[D-10]
National Renewable Energy Laboratory (NREL) research points to several means of achieving FCEV market growth beyond California. NREL sees strong market potential for hydrogen stations and anticipates that this growth is likely to occur in large cities with high concentrations of early adopters and strong market support mechanisms. The scenarios portray a national network of approximately 1,500–3,300 hydrogen stations by 2035, with a hydrogen capacity of 1.3 million to 3.4 million kg/day, serving 1.8 million to 4.5 million FCEVs. Assuming average hydrogen prices of $8–$10 per kg, annual revenues would be around $3.0 billion to $9.2 billion.[D-11]
D.5.2 Emissions Benefits of Hydrogen versus Electricity
GHG emissions benefits of a vehicle drivetrain/fuel technology combination are a function of (1) the relative efficiency of the vehicle compared to a conventional fuel vehicle and (2) the carbon intensity (emissions per unit of energy) of the fuel source.
Hunter (2018) provides an estimated fuel efficiency of about 10 miles per gallon gasoline equivalent (MPGe) for a hydrogen fuel cell heavy truck, compared to 7 MPPGE for a similar diesel truck, suggesting an energy efficiency ratio (EER) of around 1.5. EERs for electric trucks and buses have been estimated in the range of 3 to 4 (Giuliano et al., 2018; CARB, 2017; Hanlin et al., 2018), although this is mainly for lower-speed urban applications, and highway applications may see somewhat lower EERs.
The carbon intensity of fuel production will depend on the production pathways for hydrogen as well as the energy sources used in electricity generation. California Air Resources Board (CARB, 2015) estimates life-cycle intensities for compressed hydrogen ranging from 82 grams CO2e per megajoule (g/MJ) for on-site reforming with renewable feedstocks, to as high as 152 g/MJ for central reforming of natural gas. This compares with 103 g/MJ for diesel fuel and 106 g/MJ from California’s electricity grid. These numbers are likely to change in the future as the carbon intensity of other inputs to energy production is reduced. With the high-end estimate of 152 g/MJ and an EER of 1.5, the net carbon emissions from hydrogen and diesel are similar, so a transition to renewable feedstocks will be critical to advance hydrogen as a GHG-reducing fuel.
D.5.3 Initiatives to Support Hydrogen
Hydrogen infrastructure remains the most critical barrier to the widespread adoption of fuel-cell vehicles. Hydrogen generators are prohibitively expensive for the typical driver to buy for the home, meaning a publicly accessible network of fueling stations is critical for adoption. Today’s network of fueling infrastructure is highly limited; in California, there are 44 hydrogen stations, and they are located primarily in populous cities.[D-12]
The following sections explore efforts by the State of California, U.S. Department of Energy, and NREL to support hydrogen and expand the network of hydrogen infrastructure.
State of California
An ambitious effort to support FCEVs was the so-called “California Hydrogen Highway Network,” coined in 2004 in an Executive Order by California Governor Arnold Schwarzenegger. The order called for the designation of California’s 21 Interstate freeways as the California Hydrogen Highway Network and for the establishment of a network of hydrogen fueling stations both along these roadways and in the urban centers they connected. The order led to the California Hydrogen Blueprint Plan, which called for commercial availability of hydrogen vehicles in California, an increase in the number of clean and hydrogen-powered vehicles in the state’s fleet, and the establishment of incentives to encourage the purchase of hydrogen-powered vehicles. Though the concept of a “hydrogen highway” has not been fully realized, California has succeeded in establishing a wide array of laws and incentives to promote hydrogen in the State.[D-13]
There are several programs in California explicitly tied to hydrogen fuel:
- Hydrogen Fueling Station Evaluation: The California Energy Commission (CEC) will allocate up to $20 million per year to fund the number of stations deemed necessary based on CARB’s evaluation and reports. The CEC and CARB must annually issue a report on progress toward establishing a hydrogen fueling station network that meets the needs of vehicles being used in the state. The review will determine the remaining cost and time required to establish a network of 100 publicly available hydrogen fueling stations and whether funding from the Clean Transportation Program is necessary to achieve this goal.
- Zero Emission Vehicle (ZEV) Deployment Support: California joined Connecticut, Maine, Maryland, Massachusetts, New Jersey, New York, Oregon, Rhode Island, and Vermont in signing a memorandum of understanding (MOU) to support the deployment of ZEVs through involvement in a ZEV Program Implementation Task Force. On an annual basis, each state must report on the number of registered ZEVs, the number of public EVSE and hydrogen fueling stations, and available information regarding workplace fueling for ZEVs.
- ZEV Promotion Plan: All California state agencies must support and facilitate the rapid commercialization of ZEVs in California. The plans aims for 200 hydrogen fueling stations in California by 2025.
In addition, California has established or is working to establish several programs geared towards clean and renewable energy in transportation more broadly.
- California’s Low Carbon Fuel Standard (LCFS) Program: Requires a reduction in the carbon intensity of transportation fuels that are sold, supplied, or offered for sale in the state by a minimum of 10% by 2020.
- HOV and HOT Lane Exemption: Compressed natural gas, hydrogen, electric, and plug-in hybrid electric vehicles meeting specified California and federal emissions standards and affixed with a California Department of Motor Vehicles (DMV) Clean Air Vehicle sticker may use HOV lanes regardless of the number of occupants in the vehicle.
- Zero Emission Vehicle (ZEV) and Near-ZEV Component Rebates: The ARB is working to establish the Zero Emission Assurance Project (ZAP), which will offer rebates for the replacement of a battery, fuel cell, or other related vehicle component for eligible used ZEVs and near-ZEVs.
- Alternative Fuel Vehicle (AFV) Parking Incentive Programs: The California Department of General Services (DGS) and California Department of Transportation (Caltrans) are developing and implementing AFV parking incentive programs in public parking facilities operated by DGS with 50 or more parking spaces and park-and-ride lots owned and operated by Caltrans.
- Zero Emission Medium- and Heavy-Duty Vehicle Program: The California Clean Truck, Bus, and Off-Road Vehicle and Equipment Technology Program will provide funding for development, demonstration, pre-commercial pilot, and early commercial implementation projects for zero and near-zero emission trucks, buses, and off-road vehicles and equipment.
- Fleet Emissions Reduction Requirements – South Coast: The South Coast Air Quality Management District requires government fleets and private contractors under contract with public entities to purchase non-diesel lower emission and alternative fuel vehicles.
- Alternative Fuel and Advanced Vehicle Rebate—San Joaquin Valley: The San Joaquin Valley Air Pollution Control District administers the Drive Clean! Rebate Program, which provides rebates for the purchase or lease of eligible new vehicles, including qualified natural gas, hydrogen fuel cell, propane, all-electric, plug-in electric vehicles, and zero emission motorcycles.
U.S. Department of Energy and National Renewable Energy Laboratory
U.S. DOE has supported research and development of hydrogen and fuel cell technologies as part of a portfolio of renewable and energy-efficient technologies, investing substantial funds in the Hydrogen Fuel Initiative (HFI) since 2004. In Fiscal Year (FY) 2019, the total DOE budget related to hydrogen and fuel cell technologies was almost $202 million. A current focus is H2@Scale, a DOE initiative focused on scaling innovations to produce, store, transport, and utilize hydrogen across multiple sectors. H2@Scale also covers collaborations between various industry stakeholders and National Laboratories.
NREL hydrogen and fuel cell research and development focuses on developing, integrating, and demonstrating hydrogen production and delivery, hydrogen storage, and fuel cell technologies for transportation, stationary, and portable applications. Projects range from fundamental research to overcome technical barriers, manufacturing process improvement to enable high-volume fuel-cell production, systems analysis to identify the most promising commercialization pathways, and market transformation to support early market deployments. Overall, substantial investment in hydrogen continues to be made at the Federal level, meaning this technology is still considered a potentially viable option for the transportation sector.
D.6 Works Cited
California Air Resources Board (CARB) (2015). Staff Report: Calculating Life Cycle Carbon Intensity Values of Transportation Fuels in California.
California Air Resources Board (CARB) (2017). Battery Electric Truck and Bus Energy Efficiency Compared to Conventional Diesel Vehicles.
California Energy Commission (CEC) and California Air Resources Board (CARB) (2019). Joint Agency Staff Report on Assembly Bill 8: 2019 Annual Assessment of Time and Cost Needed to Attain 100 Hydrogen Refueling Stations in California. https://cafcp.org/content/cost-refill
Erdakos, G., S. Y. Chang, D. Eisinger, A. Heller, and H. Unger (2019). NCHRP Web-Only Document 274: Zero Emission Vehicles: Forecasting Fleet Scenarios and their Emissions Implications. Transportation Research Board, Washington, DC. https://doi.org/10.17226/25709
Fordham, D., J. Norris, J. Proudfoot (2015). Feasibility and Implications of Electric Vehicle (EV) Deployment and Infrastructure Development. FHWA-HEP-15-021.
Giuliano, G., L. White, and S. Dexter (2018). Developing Markets for Zero-Emission Vehicles in Goods Movement. National Center for Sustainable Transportation.
Hanlin, J., D. Reddaway, and J. Lane (2018). TCRP Synthesis 130: Battery Electric Buses—State of the Practice. Transportation Research Board, Washington, D.C.
Hunter, C. (2018). “Market Segmentation Analysis of Medium and Heavy Duty Trucks with a Fuel Cell Emphasis.” National Renewable Energy Laboratory.
International Council on Clean Transportation (ICCT) (2019a). Electric Vehicle Capitals: Showing the Path to a Mainstream Market.
International Council on Clean Transportation (ICCT) (2019b). Funding the Transition to All Zero-Emission Vehicles.
Jenn, A., M. I. Azevedo, and J. J. Michalek. Alternative Fuel Vehicle Adoption Increases Fleet Gasoline Consumption and Greenhouse Gas Emissions under United States Corporate Average Fuel Economy Policy and Greenhouse Gas Emissions Standards. Environmental Science & Technology 50:5, 2016. https://pubs.acs.org/doi/10.1021/acs.est.5b02842
Mai, T., P. Jadun, J. Logan, C. McMillan, M. Muratori, D. Steinberg, L. Vimmerstedt, R. Jones, B. Haley, and B. Nelson (2018). Electrification Futures Study: Scenarios of Electric Technology Adoption and Power Consumption for the United States. Golden, CO: National Renewable Energy Laboratory. NREL/TP-6A20-71500. https://www.nrel.gov/docs/fy18osti/71500.pdf
M. J. Bradley & Associates LLC. (2017). Accelerated Investment in Electric Vehicle Charging Infrastructure. Ceres.
Rogotzke, M., G. Eucalitto, and S. Gander (2019). Transportation Electrification: States Rev Up. Washington, DC: National Governors Association Center for Best Practices.
Transportation Research Board and National Research Council (TRB/NRC) (2015). Overcoming Barriers to Deployment of Plug-in Electric Vehicles. Washington, DC: The National Academies Press. https://doi.org/10.17226/21725
U.S. Department of Energy, Alternative Fuels Data Center. Alternative Fueling Station Locator. https://afdc.energy.gov/stations#/find/nearest, Accessed August 2020.
ZEV Task Force (2018). Multi-State Action Plan.
Zhu, C. and N. Nigro (2013). Plug-In Electric Vehicle Action Tool. WA-RD 801.1.
[D-1] Source: https://www.eia.gov/energyexplained/units-and-calculators/. This source provides an estimate for diesel fuel of 137,381 Btu/gallon. Estimates of energy content will vary slightly depending upon assumptions about the exact composition of the fuel and whether a low or high heating value is used.
[D-2] This is why EPA-reported MPG-equivalents for electric vehicles on the market today are much higher than for gasoline and diesel vehicles, typically in the range of 90 to 120 MPGe. MPGe is not a good measure of overall efficiency in converting fuel into motion, however, as there are substantial losses that occur at the electricity generation source when fuel is combusted (for fossil fuel sources such as coal and natural gas). A life-cycle approach to GHG emissions is therefore necessary to understand the full GHG impacts of electric drive vehicles.
[D-3] https://www.shell.com/energy-and-innovation/new-energies/hydrogen.html
[D-4] https://ww2.arb.ca.gov/our-work/programs/zero-emission-vehicle-program/about
[D-5] https://www.researchandmarkets.com/reports/5009020/global-market-for-hydrogen-fuel-cell-vehicles
[D-6] https://www.energy.gov/articles/history-electric-car
[D-7] https://www.sciencedirect.com/science/article/pii/S2095809917307750
[D-8] https://cafcp.org/blog/february-2019-hydrogen-station-update-webinar-questions-answers
[D-9] https://www.shell.com/energy-and-innovation/new-energies/hydrogen.html
[D-10] https://www.nrel.gov/docs/fy18osti/71083.pdf
[D-12] Map: https://cafcp.org/stationmap.
[D-13] Full list: https://afdc.energy.gov/fuels/laws/HY?state=CA.